The difference between analog and digital modulation is as follows:
Analog modulation
1. In analog modulation ,the modulated (message) signal is continuous both in amplitude and time.
2. In this modulation, the transmission of data in analog format and output also analog in nature
3. In analog modulation, any value between maximum and minimum is valid.
4. Analog modulation is more preferable than digital in Case of transmission of data, a voice signal can directly transmitted through analog.
5. It is more sensitive to noise.
6. Implementation of analog modulation is cheaper.
7. It gives less accurate results.
HDMI Encoder Modulator,16in1 Digital Headend, HD RF Modulator at SOUKA https://www.soukacatv.com/.
Digital modulation
1. In digital modulation, the modulated(message)signal is discrete both in time and amplitude
2. Transmission of the data in digital format in terms of 1’s and 0’s and output also digital in nature.
3. In digital modulation only 2 values, one value is 0 and another is 1 and remaining values are considered as noise and are rejected
4. In case of transmission of any signal digital modulation is less preferable. for example if you considered a voice signal, transmission of voice signal through digital modulation is very difficult first we need to pass it through an ADC(analog to digital converter ) at transmitter side and DAC(digital to analog converter) at receivers side to receive the original signal .
5. Less sensitive to noise , ease of multiplexing
6. Digital modulation implementation is costly than analog
7. It Produces more accurate output than analog.
Why is modulation used in communication?
The message signal frequency is raised in the modulation technique with respect to a range that is very useful for transmission. Let us see few points to describe the modulation’s significance in a communication system.
In signal transmission, the signals from various sources are transmitted with the common channel along with the usage of multiplexers. At a particular bandwidth if the signals are transmitted then they lead to interference.
There are lots of career frequencies that are modulated by the speech signals to overcome the interference.
There is one more technical reason which is nothing but the antenna size which is inversely proportional to the radiated signal frequency. 1/10th of the signal wavelength is the order of the antenna aperture size. If the signal frequency is 5 kHz then the size is practicable. Thus it is significant to increase the frequency by the process of modulation that will actually decrease the antenna height.
It is very significant to transfer the signals for longer distances termed Modulation as it is not possible to send low-frequency signals for longer distances.
It is very significant to allocate lots of channels for users and to enhance the noise immunity
Different Types of Modulation
There are two types of modulation and they are analog and digital modulation techniques. The information of the baseband is converted into Radio Frequency signals in both the techniques but then in analog modulation, these RF communication signals are having a continuous range of values which is not the case in digital as it contains discrete states.
Analog Modulation
The carrier wave in this signal is a continuously changing sine wave which in turn changes the data signal or message signal. This wave contains three general functions like amplitude, frequency, and phase.
Established in 2000, the Soukacatv.com (DSW) main products are modulators both in digital and analog modulators, amplifier and combiner. We are the leading communication supplier in manufacturing the headend system in China. Our 16 in 1 and 24 in 1 now are the most popular products all over the world. For more, please access to https://www.soukacatv.com/.
Source: quora
2018年12月28日星期五
2018年12月26日星期三
Basic Consideration about Digital modulation | Soukacatv.com
The first step in
transmitting radio-frequency signals through space is to create a
pure carrier at the transmitter. This electrical wave must be of stable,
unvarying amplitude, frequency and phase. A quartz crystal oscillator
frequently serves the purpose. Information can be conveyed when the carrier is
modulated. At the receiver, the carrier is demodulated, separating out an audio
or another signal.
It is also possible, using the common super-heterodyne
principle, to alter the carrier frequency. The idea uses frequency mixing to
convert a received signal to a fixed intermediate frequency (IF) which can be
more conveniently processed than the original radio carrier frequency. For each
frequency that is broadcast, a different beat frequency is required if a consistent
IF is to result.
HDMI Encoder
Modulator,16in1 Digital Headend, HD RF Modulator at SOUKA https://www.soukacatv.com/.
To this end, in the
early years of radio, broadcasters sent a heterodyne frequency that they
generated alongside the primary broadcast carrier. Soon it was found to be more
efficient to generate the beat frequency locally, i.e. at the receiver, and
that is how it is done currently. That is why the traditional variable
capacitor was two-gang, with twin sets of plates on the same shaft. As
different stations were tuned in, the receiver created the appropriate beat
frequency. Today essentially the same process takes place in an electronic
tuner.
In various modulation schemes, any readable
alteration of the carrier’s signal qualities can suffice to carry
information. The usual parameters are amplitude, frequency and phase. These can
be used singly or in combination, and they are applicable to both analog and
digital modulation. A previous article discussed analog modulation. Now we’ll
take a closer look at digital modulation.
Digital modulation modifies an analog carrier
signal with another discrete signal. Digital modulation methods can be
considered digital-to-analog conversion and the corresponding demodulation or
detection analog-to-digital conversion. Digital transmission has significant
advantages over its analog counterpart. For one thing, it is relatively noise
free. If noise is introduced, it originates outside of the digital transmission
proper, that is in the pre-modulation and post-demodulation zones. Furthermore,
digital modulation makes better use of bandwidth. As there is increasing
competition for limited spectrum, this characteristic assumes ever-greater
importance.
The standard method for representing digital
modulation is by means of a polar diagram. This display represents amplitude
and phase in a single graphic. The length of a vector arrow corresponds to the
magnitude and the angle of that line with respect to a horizontal line
connecting the origin to the zero-degree point on the circle represents the
phase angle that is invoked in the modulation process. Amplitude and phase
modulation are used in concert to convey the digital information. Any
instantaneous value of the digital signal can be represented by a point within
the polar diagram.
Digital modulation is generally represented
by making reference to I and Q. The I axis is the zero-degree horizontal
reference line on the polar diagram. The Q axis is the vertical line that
represents a 90° polarization angle.
The vector in the polar
diagram represents an RF carrier with an output power represented by the length
of the vector and a certain phase angle represented by the angle with the
horizontal axis. If the RF carrier has a constant output power, it could be represented
on the polar diagram as a vector with a constant length (amplitude) which
follows the trajectory of a circle.
Phase and magnitude fluctuations taken
together convey the digital information. The result is expressed in terms of I
and Q where the signal vector’s projection onto the I axis lies on the zero
degrees reference (thus called the In-phase component) and the projection onto
the Q axis lies on the 90° shifted phase reference (Quadrature component). The
phase and amplitude information of the signal, often called S(t), with a
carrier frequency w is then expressed in terms of I and Q by the equation:
S(t) = I(t)cos(w)+Q(t)sin(w)
Recall from algebra that the sine and cosine are 90° out
of phase with each other. That relationship leads to the basic topology of a
digital modulator or demodulator.
For a transmitter, I and
Q data are applied at the inputs of two different mixers driven by a local
oscillator (or RF carrier) frequency of w. The local oscillator is shifted by
90° before it drives the mixer for the Q data. The mixers form the
multiplication of the terms given in the equation.
Nearly every digital modulator or demodulator
uses this principle. Data to be transmitted gets coded into I/Q pairs before
being fed to an I/Q modulator. The necessary circuitry can be built with
digital logic or programmed in a DSP. Moreover, at a cost of greater
complexity, the I-Q modulation scheme is more bandwidth-efficient than analog
modulation.
Established
in 2000, the Soukacatv.com (DSW) main products are modulators both in digital
and analog modulators, amplifier and combiner. We are the leading communication
supplier in manufacturing the headend system in China. Our 16 in 1 and 24 in 1
now are the most popular products all over the world. For more, please access
to https://www.soukacatv.com/.
Source: testandmeasurementtips
2018年12月25日星期二
How to Understand the Modern Digital Modulation Techniques? | Soukacatv.com
Fundamental to all wireless communications is modulation, the process of impressing the data to be transmitted on the radio carrier. Most wireless transmissions today are digital, and with the limited spectrum available, the type of modulation is more critical than it has ever been.
The main goal of modulation today is to squeeze as much data into the least amount of spectrum possible. That objective, known as spectral efficiency, measures how quickly data can be transmitted in an assigned bandwidth. The unit of measurement is bits per second per Hz (b/s/Hz). Multiple techniques have emerged to achieve and improve spectral efficiency.
HDMI Encoder Modulator,16in1 Digital Headend,HD RF Modulator at SOUKA https://www.soukacatv.com/.
Amplitude Shift Keying (ASK) and Frequency Shift Keying (FSK)
There are three basic ways to modulate a sine wave radio carrier: modifying the amplitude, frequency, or phase. More sophisticated methods combine two or more of these variations to improve spectral efficiency. These basic modulation forms are still used today withdigital signals.
1. Three basic digital modulation formats are still very popular with low-data-rate short-range wireless applications: amplitude shift keying (a), on-off keying (b), and frequency shift keying (c). These waveforms are coherent as the binary state change occurs at carrier zero crossing points.
Figure 1 shows a basic serial digital signal of binary zeros and ones to be transmitted and the corresponding AM and FM signals resulting from modulation. There are two types of AM signals: on-off keying (OOK) and amplitude shift keying (ASK). In Figure 1a, the carrier amplitude is shifted between two amplitude levels to produce ASK. In Figure 1b, the binary signal turns the carrier off and on to create OOK.
AM produces sidebands above and below the carrier equal to the highest frequency content of the modulating signal. The bandwidth required is two times the highest frequency content including any harmonics for binary pulse modulating signals.
Frequency shift keying (FSK) shifts the carrier between two different frequencies called the mark and space frequencies, or fm and fs(Fig. 1c). FM produces multiple sideband frequencies above and below the carrier frequency. The bandwidth produced is a function of the highest modulating frequency including harmonics and the modulation index, which is:
m = Δf(T)
Δf is the frequency deviation or shift between the mark and space frequencies, or:
Δf = fs – fm
T is the bit time interval of the data or the reciprocal of the data rate (1/bit/s).
Smaller values of m produce fewer sidebands. A popular version of FSK called minimum shift keying (MSK) specifies m = 0.5. Smaller values are also used such as m = 0.3.
Here are two ways to further improve the spectral efficiency for both ASK and FSK. First, select data rates, carrier frequencies, and shift frequencies so there are no discontinuities in the sine carrier when changing from one binary state to another. These discontinuities produce glitches that increase the harmonic content and the bandwidth.
The idea is to synchronize the stop and start times of the binary data with when the sine carrier is transitioning in amplitude or frequency at the zero crossing points. This is called continuous phase or coherent operation. Both coherent ASK/OOK and coherent FSK have fewer harmonics and a narrower bandwidth than non-coherent signals.
A second technique is to filter the binary data prior to modulation. This rounds the signal off, lengthening the rise and fall times and reducing the harmonic content. Special Gaussian and raised cosine low pass filters are used for this purpose. GSM cell phones widely use a popular combination, Gaussian filtered MSK (GMSK), which allows a data rate of 270 kbits/s in a 200-kHz channel.
Binary Phase Shift Keying (BPSK) And Quadrature Phase Shift Keying (QPSK)
A very popular digital modulation scheme, binary phase shift keying (BPSK), shifts the carrier sine wave 180° for each change in binary state (Fig. 2). BPSK is coherent as the phase transitions occur at the zero crossing points. The proper demodulation of BPSK requires the signal to be compared to a sine carrier of the same phase. This involves carrier recovery and other complex circuitry.
2. In binary phase shift keying, note how a binary 0 is 0° while a binary 1 is 180°. The phase changes when the binary state switches so the signal is coherent.
A simpler version is differential BPSK or DPSK, where the received bit phase is compared to the phase of the previous bit signal. BPSK is very spectrally efficient in that you can transmit at a data rate equal to the bandwidth or 1 bit/Hz.
In a popular variation of BPSK, quadrature PSK (QPSK), the modulator produces two sine carriers 90° apart. The binary data modulates each phase, producing four unique sine signals shifted by 45° from one another. The two phases are added together to produce the final signal. Each unique pair of bits generates a carrier with a different phase (Table 1).
Figure 3a illustrates QPSK with a phasor diagram where the phasor represents the carrier sine amplitude peak and its position indicates the phase. A constellation diagram in Figure 3b shows the same information. QPSK is very spectrally efficient since each carrier phase represents two bits of data. The spectral efficiency is 2 bits/Hz, meaning twice the data rate can be achieved in the same bandwidth as BPSK.
3. Modulation can be represented without time domain waveforms. For example, QPSK can be represented with a phasor diagram (a) or a constellation diagram (b), both of which indicate phase and amplitude magnitudes.
Data Rate And Baud RateThe maximum theoretical data rate or channel capacity (C) in bits/s is a function of the channel bandwidth (B) channel in Hz and the signal-to-noise ratio (SNR):
C = B log2 (1 + SNR)
This is called the Shannon-Hartley law. The maximum data rate is directly proportional to the bandwidth and logarithmically proportional the SNR. Noise greatly diminishes the data rate for a given bit error rate (BER).
Another key factor is the baud rate, or the number of modulation symbols transmitted per second. The term symbol in modulation refers to one specific state of a sine carrier signal. It can be an amplitude, a frequency, a phase, or some combination of them. Basic binary transmission uses one bit per symbol.
In ASK, a binary 0 is one amplitude and a binary 1 is another amplitude. In FSK, a binary 0 is one carrier frequency and a binary 1 is another frequency. BPSK uses a 0° shift for a binary 0 and a 180° shift for a binary 1. In each of these cases there is one bit per symbol.
Data rate in bits/s is calculated as the reciprocal of the bit time (tb):
bits/s = 1/tb
With one symbol per bit, the baud rate is the same as the bit rate. However, if you transmit more bits per symbol, the baud rate is slower than the bit rate by a factor equal to the number of bits per symbol. For example, if 2 bits per symbol are transmitted, the baud rate is the bit rate divided by 2. For instance, with QPSK a 70 Mb/s data stream is transmitted at a baud rate of 35 symbols/second.
Multiple Phase Shift Keying (M-PSK)
QPSK produces two bits per symbol, making it very spectrally efficient. QPSK can be referred to as 4-PSK because there are four amplitude-phase combinations. By using smaller phase shifts, more bits can be transmitted per symbol. Some popular variations are 8-PSK and 16-PSK.
8-PSK uses eight symbols with constant carrier amplitude 45° shifts between them, enabling three bits to be transmitted for each symbol. 16-PSK uses 22.5° shifts of constant amplitude carrier signals. This arrangement results in a transmission of 4 bits per symbol.
While Multiple Phase Shift Keying (M-PSK) is much more spectrally efficient, the greater the number of smaller phase shifts, the more difficult the signal is to demodulate in the presence of noise. The benefit of M-PSK is that the constant carrier amplitude means that more efficient nonlinear power amplification can be used.
Quadrature Amplitude Modulation (QAM)
The creation of symbols that are some combination of amplitude and phase can carry the concept of transmitting more bits per symbol further. This method is called quadrature amplitude modulation (QAM). For example, 8QAM uses four carrier phases plus two amplitude levels to transmit 3 bits per symbol. Other popular variations are 16QAM, 64QAM, and 256QAM, which transmit 4, 6, and 8 bits per symbol respectively (Fig. 4).
4. 16QAM uses a mix of amplitudes and phases to achieve 4 bits/Hz. In this example, there are three amplitudes and 12 phase shifts.
While QAM is enormously efficient of spectrum, it is more difficult to demodulate in the presence of noise, which is mostly random amplitude variations. Linear power amplification is also required. QAM is very widely used in cable TV, Wi-Fi wireless local-area networks (LANs), satellites, and cellular telephone systems to produce maximum data rate in limited bandwidths.
Amplitude Phase Shift Keying (APSK)
Amplitude phase shift keying (APSK), a variation of both M-PSK and QAM, was created in response to the need for an improved QAM. Higher levels of QAM such as 16QAM and above have many different amplitude levels as well as phase shifts. These amplitude levels are more susceptible to noise.
Furthermore, these multiple levels require linear power amplifiers (PAs) that are less efficient than nonlinear (e.g., class C). The fewer the number of amplitude levels or the smaller the difference between the amplitude levels, the greater the chance to operate in the nonlinear region of the PA to boost power level.
APSK uses fewer amplitude levels. It essentially arranges the symbols into two or more concentric rings with a constant phase offset θ. For example, 16APSK uses a double-ring PSK format (Fig. 5). This is called 4-12 16APSK with four symbols in the center ring and 12 in the outer ring.
5. 16APSK uses two amplitude levels, A1 and A2, plus 16 different phase positions with an offset of θ. This technique is widely used in satellites.
Two close amplitude levels allow the amplifier to operate closer to the nonlinear region, improving efficiency as well as power output. APSK is used primarily in satellites since it is a good fit with the popular traveling wave tube (TWT) PAs.
Orthogonal Frequency Division Multiplexing (OFDM)
Orthogonal frequency division multiplexing (OFDM) combines modulation and multiplexing techniques to improve spectral efficiency. A transmission channel is divided into many smaller subchannels or subcarriers. The subcarrier frequencies and spacings are chosen so they’re orthogonal to one another. Their spectra won’t interfere with one another, then, so no guard bands are required (Fig. 6).
6. In the OFDM signal for the IEEE 802.11n Wi-Fi standard, 56 subcarriers are spaced 312.5 kHz in a 20-MHz channel. Data rates to 300 Mbits/s can be achieved with 64QAM.
The serial digital data to be transmitted is subdivided into parallel slower data rate channels. These lower data rate signals are then used to modulate each subcarrier. The most common forms of modulation are BPSK, QPSK, and several levels of QAM. BPSK, QPSK, 16QAM, and 64QAM are defined with 802.11n. Data rates up to about 300 Mbits/s are possible with 64QAM.
The complex modulation process is only produced by digital signal processing (DSP) techniques. An inverse fast Fourier transform (IFFT) generates the signal to be transmitted. An FFT process recovers the signal at the receiver.
OFDM is very spectrally efficient. That efficiency level depends on the number of subcarriers and the type of modulation, but it can be as high as 30 bits/s/Hz. Because of the wide bandwidth it usually occupies and the large number of subcarriers, it also is less prone to signal loss due to fading, multipath reflections, and similar effects common in UHF and microwave radio signal propagation.
Currently, OFDM is the most popular form of digital modulation. It is used in Wi-Fi LANs, WiMAX broadband wireless, Long Term Evolution (LTE) 4G cellular systems, digital subscriber line (DSL) systems, and in most power-line communications (PLC) applications. For more, see “Orthogonal Frequency-Division Multiplexing (OFDM): FAQ Tutorial.”
Determining Spectral Efficiency
Again, spectral efficiency is a measure of how quickly data can be transmitted in an assigned bandwidth, and the unit of measurement is bits/s/Hz (b/s/Hz). Each type of modulation has a maximum theoretical spectral efficiency measure (Table 2).
SNR is another important factor that influences spectral efficiency. It also can be expressed as the carrier to noise power ratio (CNR). The measure is the BER for a given CNR value. BER is the percentage of errors that occur in a given number of bits transmitted. As the noise becomes larger compared to the signal level, more errors occur.
Some modulation methods are more immune to noise than others. Amplitude modulation methods like ASK/OOK and QAM are far more susceptible to noise so they have a higher BER for a given modulation. Phase and frequency modulation (BPSK, FSK, etc.) fare better in a noisy environment so they require less signal power for a given noise level (Fig. 7).
7. This is a comparison of several popular modulation methods and their spectral efficiency expressed in terms of BER versus CNR. Note that for a given BER, a greater CNR is needed for the higher QAM levels.
Other Factors Affecting Spectral Efficiency
While modulation plays a key role in the spectral efficiency you can expect, other aspects in wireless design influence it as well. For example, the use of forward error correction (FEC) techniques can greatly improve the BER. Such coding methods add extra bits so errors can be detected and corrected.
These extra coding bits add overhead to the signal, reducing the net bit rate of the data, but that’s usually an acceptable tradeoff for the single-digit dB improvement in CNR. Such coding gain is common to almost all wireless systems today.
Digital compression is another useful technique. The digital data to be sent is subjected to a compression algorithm that greatly reduces the amount of information. This allows digital signals to be reduced in content so they can be transmitted as shorter, slower data streams.
For example, voice signals are compressed for digital cell phones and voice over Internet protocol (VoIP) phones. Music is compressed in MP3 or AAC files for faster transmission and less storage. Video is compressed so high-resolution images can be transmitted faster or in bandwidth-limited systems.
Another factor affecting spectral efficiency is the use of multiple-input multiple-output (MIMO), which is the use of multiple antennas and transceivers to transmit two or more bit streams. A single high-rate stream is divided into two parallel streams and transmitted in the same bandwidth simultaneously.
By coding the streams and their unique path characteristics, the receiver can identify and demodulate each stream and reassemble it into the original stream. MIMO, therefore, improves data rate, noise performance, and spectral efficiency. Newer wireless LAN (WLAN) standards like 802.11n and 802.11ac/ad and cellular standards like LTE and WiMAX use MIMO. For more, see “How MIMO Works.”
Implementing Modulation And Demodulation
In the past, unique circuits implemented modulation and demodulation. Today, most modern radios are software-defined radios (SDR) where functions like modulation and demodulation are handled in software. DSP algorithms do the job previously assigned to modulator and demodulator circuits.
The modulation process begins with the data to be transmitted being fed to a DSP device that generates two digital outputs, which are needed to define the amplitude and phase information required at the receiver to recover the data. The DSP produces two baseband streams that are sent to digital-to-analog converters (DACs) that produce the analog equivalents.
These modulation signals feed the mixers along with the carrier. There is a 90° shift between the carrier signals to the mixers. The resulting quadrature output signals from the mixers are summed to produce the signal to be transmitted. If the carrier signal is at the final transmission frequency, the composite signal is ready to be amplified and sent to the antenna. This is called direct conversion. Alternately, the carrier signal may be at a lower intermediate frequency (IF). The IF signal is upconverted to the final carrier frequency by another mixer before being applied to the transmitter PA.
At the receiver, the signal from the antenna is amplified and downconverted to IF or directly to the original baseband signals. The amplified signal from the antenna is applied to mixers along with the carrier signal. Again, there is a 90° shift between the carrier signals applied to the mixers.
The mixers produce the original baseband analog signals, which are then digitized in a pair of analog-to-digital converters (ADCs) and sent to the DSP circuitry where demodulation algorithms recover the original digital data.
There are three important points to consider. First, the modulation and demodulation processes use two signals in quadrature with one another. The DSP calculations call for two quadrature signals if the phase and amplitude are to be preserved and captured during modulation or demodulation.
Second, the DSP circuitry may be a conventional programmable DSP chip or may be implemented by fixed digital logic implementing the algorithm. Fixed logic circuits are smaller and faster and are preferred for their low latency in the modulation or demodulation process.
Third, the PA in the transmitter needs to be a linear amplifier if the modulation is QPSK or QAM to faithfully reproduce the amplitude and phase information. For ASK, FSK, and BPSK, a more efficient non-linear amplifier may be used.
The Pursuit Of Greater Spectral Efficiency
With spectrum being a finite entity, it is always in short supply. The Federal Communications Commission (FCC) and other government bodies have assigned most of the electromagnetic frequency spectrum over the years, and most of that is actively used.
Shortages now exist in the cellular and land mobile radio sectors, inhibiting the expansion of services such as high data speeds as well as the addition of new subscribers. One approach to the problem is to improve the efficiency of usage by squeezing more users into the same or less spectrum and achieving higher data rates. Improved modulation and access methods can help.
One of the most crowded areas of spectrum is the land mobile radio (LMR) and private mobile radio (PMR) spectrum used by the federal government, state governments, and local public safety agencies like fire and police departments. Currently they’re assigned spectrum by FCC license in the 150- to 174-MHz VHF spectrum and the 421- to 512-MHz UHF spectrum.
Most radio systems and handsets use FM analog modulation that occupies a 25-kHz channel. Recently the FCC has required all such radios to switch over to 12.5-kHz channels. This conversion, known as narrowbanding, doubles the number available channels.
Narrowbanding is expected to improve a radio’s ability to get access to a channel. It also means that more radios can be added to the system. This conversion must take place before January 1, 2013. Otherwise, an agency or business could lose its license or be fined. This switchover will be expensive as new radio systems and handsets are required.
In the future, the FCC is expected to mandate a further change from the 12.5-kHz channels to 6.25-kHz channels, again doubling capacity without increasing the amount of spectrum assigned. No date for that change has been assigned.
The new equipment can use either analog or digital modulation. It is possible to put standard analog FM in a 12.5-kHz channel by adjusting the modulation index and using other bandwidth-narrowing techniques. However, analog FM in a 6.25-kHz channel is unworkable, so a digital technique must be used.
Digital methods digitize the voice signal and use compression techniques to produce a very low-rate serial digital signal that can be modulated into a narrow band. Such digital modulation techniques are expected to meet the narrowbanding goal and provide some additional performance advantages.
New modulation techniques and protocols—including P25, TETRA, DMR, dPMR, and NXDN—have been developed to meet this need. All of these new methods must meet the requirements of the FCC’s Part 90 regulations and/or the regulations of the European Telecommunications Standards Institute (ETSI) standards such as TS-102 490 and TS-102-658 for LMR.
The most popular digital LMR technology, P25, is already in wide use in the U.S. with 12.5-kHz channels. Its frequency division multiple access (FDMA) method divides the assigned spectrum into 6.25-kHz or 12.5-kHz channels.
Phase I of the P25 project uses a four-symbol FSK (4FSK) modulation. Standard FSK, covered earlier, uses two frequencies or “tones” to achieve 1-bit/Hz. However, 4FSK is a variant that uses four frequencies to provide 2-bits/Hz efficiency. With this scheme the standard achieves a 9600-bit/s data rate in a 12.5-KHz channel. With 4FSK, the carrier frequency is shifted by ±1.8 kHz or ±600 Hz to achieve the four symbols.
In Phase 2, a compatible QPSK modulation scheme is used to achieve a similar data rate in a 6.25-kHz channel. The phase is shifted either ±45° or ±135° to get the four symbols. A unique demodulator has been developed to detect either the 4FSK or QPSK signal to recover the digital voice. Only different modulators on the transmit end are needed to make the transition from Phase 1 to Phase 2.
The most widespread digital LMR technology outside of the U.S. is TETRA, or Terrestrial Trunked Radio. This ETSI standard is universally used in Europe as well as in Africa, Asia, and Latin America. Its time division multiple access (TDMA) approach multiplexes four digital voice or data signals into a 25-kHz channel.
A single channel is used to support a digital stream of four time slots for the digital data for each subscriber. This is equivalent to four independent signals in adjacent 6.25-kHz channels. The modulation is π/4-DQPSK, and the data rate is 7.2 kbits/s per time slot.
Another ETSI standard, digital mobile radio (DMR), uses a 4FSK modulation scheme in a 12.5-kHz channel. It can achieve a 6.25-kHz channel equivalent in a 12.5-kHz channel by using two-slot TDMA. The voice is digitally coded with error correction, and the basic rate is 3.6 kbits/s. The data rate in the 12.5-kHz band is 9600 kbits/s.
A similar technology is dPMR, or digital private mobile radio standard. This ETSI standard also uses a 4FSK modulation scheme, but the access is FDMA in 6.25-kHz channels. The voice coding rate is also 3.6 kbits/s with error correction.
LMR manufacturers Icom and Kenwood have developed NXDN, another standard for LMR. It is designed to operate in either 12.5- or 6.25-kHz channels using digital voice compression and a four-symbol FSK system. A channel may be selected to carry voice or data.
The basic data rate is 4800 bits/s. The access method is FDMA. NXDN and dPMR are similar, as they both use 4FSK and FDMA in 6.25-kHz channels. The two methods are not compatible, though, as the data protocols and other features are not the same.
Because all of these digital techniques are similar and operate in standard frequency ranges, Freescale Semiconductor was able to make a single-chip digital radio that includes the RF transceiver plus an ARM9 processor that can be programmed to handle any of the digital standards. The MC13260 system-on-a-chip (SoC) can form the basis of a handset radio for any one if not multiple protocols. For more, see “Chip Makes Two-Way Radio Easy.”
Another example of modulation techniques improving spectral efficiency and increasing data throughput in a given channel is a new technique from NovelSat called NS3 modulation. Satellites are positioned in an orbit around the equator about 22,300 miles from earth. This is called the geostationary orbit, and satellites in it rotate in synchronization with the earth so they appear fixed in place, making them a good signal relay platform from one place to another on earth.
Satellites carry several transponders that pick up the weak uplink signal from earth and retransmit it on a different frequency. These transponders are linear and have a fixed bandwidth, typically 36 MHz. Some of the newer satellites have 72-MHz channel transponders. With a fixed bandwidth, the data rate is somewhat fixed as determined by the modulation scheme and access methods.
The question is how one deals with the need to increase the data rate in a remote satellite as required by the ever increasing demand for more traffic capacity. The answer lies in simply creating and implementing a more spectrally efficient modulation method. That’s what NovelSat did. Its NS3 modulation method increases bandwidth capacity up to 78%.
That level of improvement comes from a revised version of APSK modulation covered earlier. One commonly used satellite transmission standard, DVB-S2, is a single carrier (typically L-band, 950 to 1750 MHz) that can use QPSK, 8PSK, 16APSK, and 32APSK modulation with different forward error correction (FEC) schemes. The most common application is video transmission.
NS3 improves on DVB-S2 by offering 64APSK with multiple amplitude and phase symbols to improve efficiency. Also included is low density parity check (LDPC) coding. This combination provides a maximum data rate of 358 Mbits/s in a 72-MHz transponder. Because the modulation is APSK, the TWT PAs don’t have to be backed off to preserve perfect linearity. As a result, they can operate at a higher power level and achieve the higher data rate with a lower CNR than DVB-S2. NovelSat offers its NS1000 modulator and NS2000 demodulator units to upgrade satellite systems to NS3. In most applications, NS3 provides a data rate boost over DVB-S2 for a given CNR.
Established in 2000, the Soukacatv.com (DSW) main products are modulators both in digital and analog modulators, amplifier and combiner. We are the leading communication supplier in manufacturing the headend system in China. Our 16 in 1 and 24 in 1 now are the most popular products all over the world. For more, please access to https://www.soukacatv.com/.
Source: electronicdesign
The main goal of modulation today is to squeeze as much data into the least amount of spectrum possible. That objective, known as spectral efficiency, measures how quickly data can be transmitted in an assigned bandwidth. The unit of measurement is bits per second per Hz (b/s/Hz). Multiple techniques have emerged to achieve and improve spectral efficiency.
HDMI Encoder Modulator,16in1 Digital Headend,HD RF Modulator at SOUKA https://www.soukacatv.com/.
Amplitude Shift Keying (ASK) and Frequency Shift Keying (FSK)
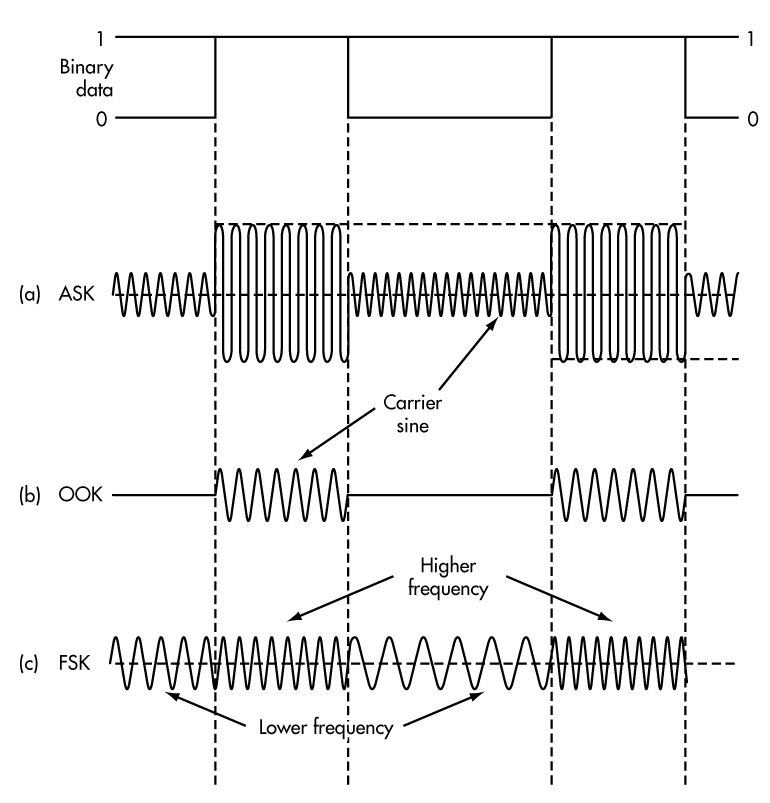
1. Three basic digital modulation formats are still very popular with low-data-rate short-range wireless applications: amplitude shift keying (a), on-off keying (b), and frequency shift keying (c). These waveforms are coherent as the binary state change occurs at carrier zero crossing points.
Figure 1 shows a basic serial digital signal of binary zeros and ones to be transmitted and the corresponding AM and FM signals resulting from modulation. There are two types of AM signals: on-off keying (OOK) and amplitude shift keying (ASK). In Figure 1a, the carrier amplitude is shifted between two amplitude levels to produce ASK. In Figure 1b, the binary signal turns the carrier off and on to create OOK.
AM produces sidebands above and below the carrier equal to the highest frequency content of the modulating signal. The bandwidth required is two times the highest frequency content including any harmonics for binary pulse modulating signals.
Frequency shift keying (FSK) shifts the carrier between two different frequencies called the mark and space frequencies, or fm and fs(Fig. 1c). FM produces multiple sideband frequencies above and below the carrier frequency. The bandwidth produced is a function of the highest modulating frequency including harmonics and the modulation index, which is:
m = Δf(T)
Δf is the frequency deviation or shift between the mark and space frequencies, or:
Δf = fs – fm
T is the bit time interval of the data or the reciprocal of the data rate (1/bit/s).
Smaller values of m produce fewer sidebands. A popular version of FSK called minimum shift keying (MSK) specifies m = 0.5. Smaller values are also used such as m = 0.3.
Here are two ways to further improve the spectral efficiency for both ASK and FSK. First, select data rates, carrier frequencies, and shift frequencies so there are no discontinuities in the sine carrier when changing from one binary state to another. These discontinuities produce glitches that increase the harmonic content and the bandwidth.
The idea is to synchronize the stop and start times of the binary data with when the sine carrier is transitioning in amplitude or frequency at the zero crossing points. This is called continuous phase or coherent operation. Both coherent ASK/OOK and coherent FSK have fewer harmonics and a narrower bandwidth than non-coherent signals.
A second technique is to filter the binary data prior to modulation. This rounds the signal off, lengthening the rise and fall times and reducing the harmonic content. Special Gaussian and raised cosine low pass filters are used for this purpose. GSM cell phones widely use a popular combination, Gaussian filtered MSK (GMSK), which allows a data rate of 270 kbits/s in a 200-kHz channel.
Binary Phase Shift Keying (BPSK) And Quadrature Phase Shift Keying (QPSK)
A very popular digital modulation scheme, binary phase shift keying (BPSK), shifts the carrier sine wave 180° for each change in binary state (Fig. 2). BPSK is coherent as the phase transitions occur at the zero crossing points. The proper demodulation of BPSK requires the signal to be compared to a sine carrier of the same phase. This involves carrier recovery and other complex circuitry.
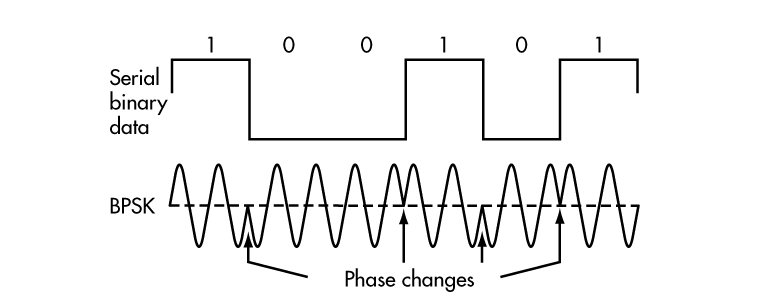
2. In binary phase shift keying, note how a binary 0 is 0° while a binary 1 is 180°. The phase changes when the binary state switches so the signal is coherent.
A simpler version is differential BPSK or DPSK, where the received bit phase is compared to the phase of the previous bit signal. BPSK is very spectrally efficient in that you can transmit at a data rate equal to the bandwidth or 1 bit/Hz.
In a popular variation of BPSK, quadrature PSK (QPSK), the modulator produces two sine carriers 90° apart. The binary data modulates each phase, producing four unique sine signals shifted by 45° from one another. The two phases are added together to produce the final signal. Each unique pair of bits generates a carrier with a different phase (Table 1).
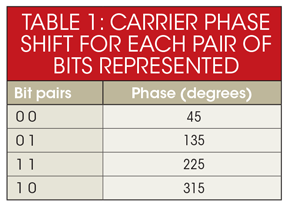
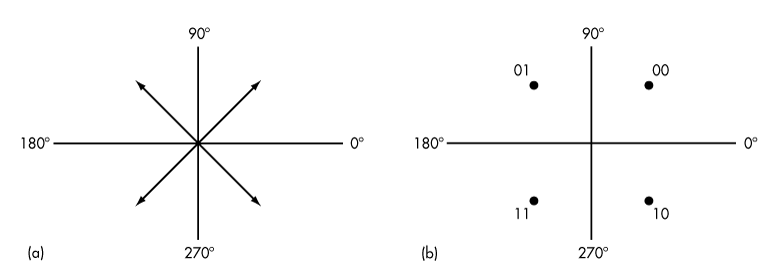
Data Rate And Baud RateThe maximum theoretical data rate or channel capacity (C) in bits/s is a function of the channel bandwidth (B) channel in Hz and the signal-to-noise ratio (SNR):
C = B log2 (1 + SNR)
This is called the Shannon-Hartley law. The maximum data rate is directly proportional to the bandwidth and logarithmically proportional the SNR. Noise greatly diminishes the data rate for a given bit error rate (BER).
Another key factor is the baud rate, or the number of modulation symbols transmitted per second. The term symbol in modulation refers to one specific state of a sine carrier signal. It can be an amplitude, a frequency, a phase, or some combination of them. Basic binary transmission uses one bit per symbol.
In ASK, a binary 0 is one amplitude and a binary 1 is another amplitude. In FSK, a binary 0 is one carrier frequency and a binary 1 is another frequency. BPSK uses a 0° shift for a binary 0 and a 180° shift for a binary 1. In each of these cases there is one bit per symbol.
Data rate in bits/s is calculated as the reciprocal of the bit time (tb):
bits/s = 1/tb
With one symbol per bit, the baud rate is the same as the bit rate. However, if you transmit more bits per symbol, the baud rate is slower than the bit rate by a factor equal to the number of bits per symbol. For example, if 2 bits per symbol are transmitted, the baud rate is the bit rate divided by 2. For instance, with QPSK a 70 Mb/s data stream is transmitted at a baud rate of 35 symbols/second.
Multiple Phase Shift Keying (M-PSK)
QPSK produces two bits per symbol, making it very spectrally efficient. QPSK can be referred to as 4-PSK because there are four amplitude-phase combinations. By using smaller phase shifts, more bits can be transmitted per symbol. Some popular variations are 8-PSK and 16-PSK.
8-PSK uses eight symbols with constant carrier amplitude 45° shifts between them, enabling three bits to be transmitted for each symbol. 16-PSK uses 22.5° shifts of constant amplitude carrier signals. This arrangement results in a transmission of 4 bits per symbol.
While Multiple Phase Shift Keying (M-PSK) is much more spectrally efficient, the greater the number of smaller phase shifts, the more difficult the signal is to demodulate in the presence of noise. The benefit of M-PSK is that the constant carrier amplitude means that more efficient nonlinear power amplification can be used.
Quadrature Amplitude Modulation (QAM)
The creation of symbols that are some combination of amplitude and phase can carry the concept of transmitting more bits per symbol further. This method is called quadrature amplitude modulation (QAM). For example, 8QAM uses four carrier phases plus two amplitude levels to transmit 3 bits per symbol. Other popular variations are 16QAM, 64QAM, and 256QAM, which transmit 4, 6, and 8 bits per symbol respectively (Fig. 4).
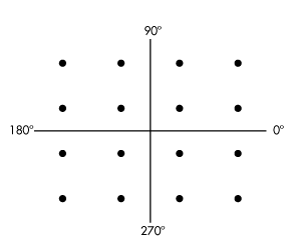
While QAM is enormously efficient of spectrum, it is more difficult to demodulate in the presence of noise, which is mostly random amplitude variations. Linear power amplification is also required. QAM is very widely used in cable TV, Wi-Fi wireless local-area networks (LANs), satellites, and cellular telephone systems to produce maximum data rate in limited bandwidths.
Amplitude Phase Shift Keying (APSK)
Amplitude phase shift keying (APSK), a variation of both M-PSK and QAM, was created in response to the need for an improved QAM. Higher levels of QAM such as 16QAM and above have many different amplitude levels as well as phase shifts. These amplitude levels are more susceptible to noise.
Furthermore, these multiple levels require linear power amplifiers (PAs) that are less efficient than nonlinear (e.g., class C). The fewer the number of amplitude levels or the smaller the difference between the amplitude levels, the greater the chance to operate in the nonlinear region of the PA to boost power level.
APSK uses fewer amplitude levels. It essentially arranges the symbols into two or more concentric rings with a constant phase offset θ. For example, 16APSK uses a double-ring PSK format (Fig. 5). This is called 4-12 16APSK with four symbols in the center ring and 12 in the outer ring.
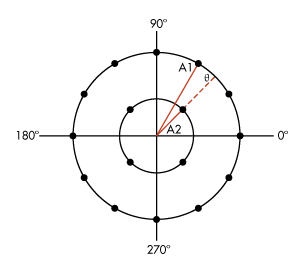
Two close amplitude levels allow the amplifier to operate closer to the nonlinear region, improving efficiency as well as power output. APSK is used primarily in satellites since it is a good fit with the popular traveling wave tube (TWT) PAs.
Orthogonal Frequency Division Multiplexing (OFDM)
Orthogonal frequency division multiplexing (OFDM) combines modulation and multiplexing techniques to improve spectral efficiency. A transmission channel is divided into many smaller subchannels or subcarriers. The subcarrier frequencies and spacings are chosen so they’re orthogonal to one another. Their spectra won’t interfere with one another, then, so no guard bands are required (Fig. 6).
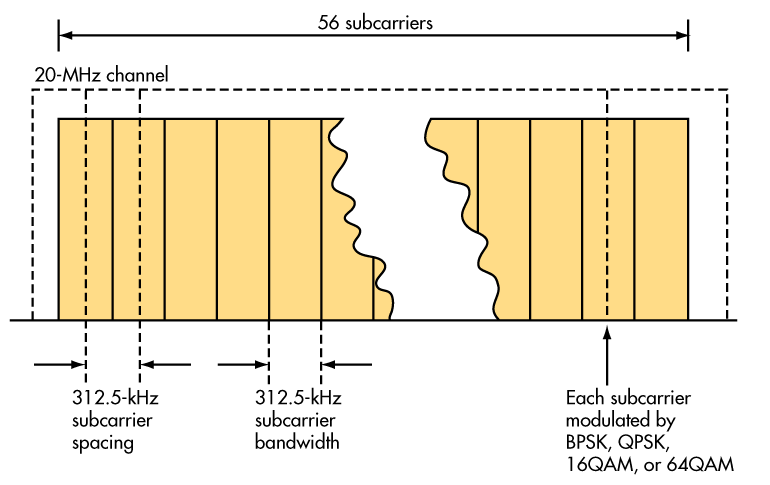
The serial digital data to be transmitted is subdivided into parallel slower data rate channels. These lower data rate signals are then used to modulate each subcarrier. The most common forms of modulation are BPSK, QPSK, and several levels of QAM. BPSK, QPSK, 16QAM, and 64QAM are defined with 802.11n. Data rates up to about 300 Mbits/s are possible with 64QAM.
The complex modulation process is only produced by digital signal processing (DSP) techniques. An inverse fast Fourier transform (IFFT) generates the signal to be transmitted. An FFT process recovers the signal at the receiver.
OFDM is very spectrally efficient. That efficiency level depends on the number of subcarriers and the type of modulation, but it can be as high as 30 bits/s/Hz. Because of the wide bandwidth it usually occupies and the large number of subcarriers, it also is less prone to signal loss due to fading, multipath reflections, and similar effects common in UHF and microwave radio signal propagation.
Currently, OFDM is the most popular form of digital modulation. It is used in Wi-Fi LANs, WiMAX broadband wireless, Long Term Evolution (LTE) 4G cellular systems, digital subscriber line (DSL) systems, and in most power-line communications (PLC) applications. For more, see “Orthogonal Frequency-Division Multiplexing (OFDM): FAQ Tutorial.”
Determining Spectral Efficiency
Again, spectral efficiency is a measure of how quickly data can be transmitted in an assigned bandwidth, and the unit of measurement is bits/s/Hz (b/s/Hz). Each type of modulation has a maximum theoretical spectral efficiency measure (Table 2).
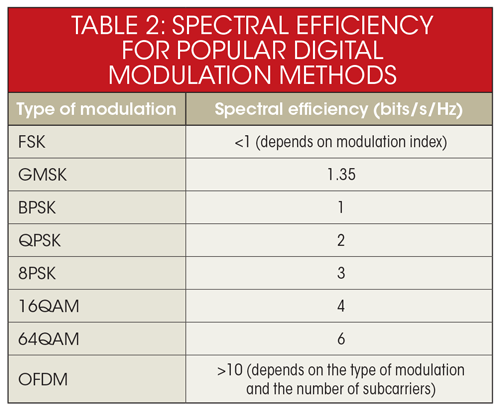
Some modulation methods are more immune to noise than others. Amplitude modulation methods like ASK/OOK and QAM are far more susceptible to noise so they have a higher BER for a given modulation. Phase and frequency modulation (BPSK, FSK, etc.) fare better in a noisy environment so they require less signal power for a given noise level (Fig. 7).
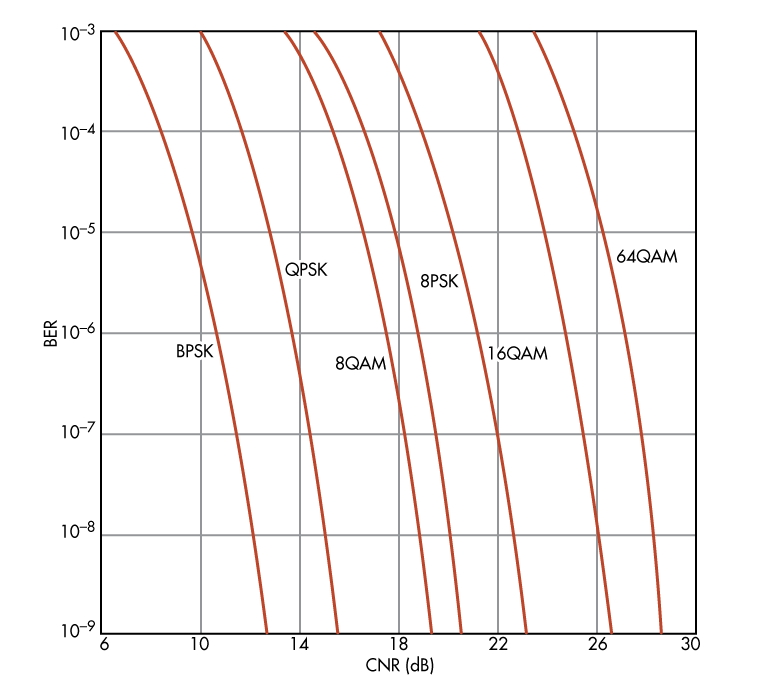
Other Factors Affecting Spectral Efficiency
While modulation plays a key role in the spectral efficiency you can expect, other aspects in wireless design influence it as well. For example, the use of forward error correction (FEC) techniques can greatly improve the BER. Such coding methods add extra bits so errors can be detected and corrected.
These extra coding bits add overhead to the signal, reducing the net bit rate of the data, but that’s usually an acceptable tradeoff for the single-digit dB improvement in CNR. Such coding gain is common to almost all wireless systems today.
Digital compression is another useful technique. The digital data to be sent is subjected to a compression algorithm that greatly reduces the amount of information. This allows digital signals to be reduced in content so they can be transmitted as shorter, slower data streams.
For example, voice signals are compressed for digital cell phones and voice over Internet protocol (VoIP) phones. Music is compressed in MP3 or AAC files for faster transmission and less storage. Video is compressed so high-resolution images can be transmitted faster or in bandwidth-limited systems.
Another factor affecting spectral efficiency is the use of multiple-input multiple-output (MIMO), which is the use of multiple antennas and transceivers to transmit two or more bit streams. A single high-rate stream is divided into two parallel streams and transmitted in the same bandwidth simultaneously.
By coding the streams and their unique path characteristics, the receiver can identify and demodulate each stream and reassemble it into the original stream. MIMO, therefore, improves data rate, noise performance, and spectral efficiency. Newer wireless LAN (WLAN) standards like 802.11n and 802.11ac/ad and cellular standards like LTE and WiMAX use MIMO. For more, see “How MIMO Works.”
Implementing Modulation And Demodulation
In the past, unique circuits implemented modulation and demodulation. Today, most modern radios are software-defined radios (SDR) where functions like modulation and demodulation are handled in software. DSP algorithms do the job previously assigned to modulator and demodulator circuits.
The modulation process begins with the data to be transmitted being fed to a DSP device that generates two digital outputs, which are needed to define the amplitude and phase information required at the receiver to recover the data. The DSP produces two baseband streams that are sent to digital-to-analog converters (DACs) that produce the analog equivalents.
These modulation signals feed the mixers along with the carrier. There is a 90° shift between the carrier signals to the mixers. The resulting quadrature output signals from the mixers are summed to produce the signal to be transmitted. If the carrier signal is at the final transmission frequency, the composite signal is ready to be amplified and sent to the antenna. This is called direct conversion. Alternately, the carrier signal may be at a lower intermediate frequency (IF). The IF signal is upconverted to the final carrier frequency by another mixer before being applied to the transmitter PA.
At the receiver, the signal from the antenna is amplified and downconverted to IF or directly to the original baseband signals. The amplified signal from the antenna is applied to mixers along with the carrier signal. Again, there is a 90° shift between the carrier signals applied to the mixers.
The mixers produce the original baseband analog signals, which are then digitized in a pair of analog-to-digital converters (ADCs) and sent to the DSP circuitry where demodulation algorithms recover the original digital data.
There are three important points to consider. First, the modulation and demodulation processes use two signals in quadrature with one another. The DSP calculations call for two quadrature signals if the phase and amplitude are to be preserved and captured during modulation or demodulation.
Second, the DSP circuitry may be a conventional programmable DSP chip or may be implemented by fixed digital logic implementing the algorithm. Fixed logic circuits are smaller and faster and are preferred for their low latency in the modulation or demodulation process.
Third, the PA in the transmitter needs to be a linear amplifier if the modulation is QPSK or QAM to faithfully reproduce the amplitude and phase information. For ASK, FSK, and BPSK, a more efficient non-linear amplifier may be used.
The Pursuit Of Greater Spectral Efficiency
With spectrum being a finite entity, it is always in short supply. The Federal Communications Commission (FCC) and other government bodies have assigned most of the electromagnetic frequency spectrum over the years, and most of that is actively used.
Shortages now exist in the cellular and land mobile radio sectors, inhibiting the expansion of services such as high data speeds as well as the addition of new subscribers. One approach to the problem is to improve the efficiency of usage by squeezing more users into the same or less spectrum and achieving higher data rates. Improved modulation and access methods can help.
One of the most crowded areas of spectrum is the land mobile radio (LMR) and private mobile radio (PMR) spectrum used by the federal government, state governments, and local public safety agencies like fire and police departments. Currently they’re assigned spectrum by FCC license in the 150- to 174-MHz VHF spectrum and the 421- to 512-MHz UHF spectrum.
Most radio systems and handsets use FM analog modulation that occupies a 25-kHz channel. Recently the FCC has required all such radios to switch over to 12.5-kHz channels. This conversion, known as narrowbanding, doubles the number available channels.
Narrowbanding is expected to improve a radio’s ability to get access to a channel. It also means that more radios can be added to the system. This conversion must take place before January 1, 2013. Otherwise, an agency or business could lose its license or be fined. This switchover will be expensive as new radio systems and handsets are required.
In the future, the FCC is expected to mandate a further change from the 12.5-kHz channels to 6.25-kHz channels, again doubling capacity without increasing the amount of spectrum assigned. No date for that change has been assigned.
The new equipment can use either analog or digital modulation. It is possible to put standard analog FM in a 12.5-kHz channel by adjusting the modulation index and using other bandwidth-narrowing techniques. However, analog FM in a 6.25-kHz channel is unworkable, so a digital technique must be used.
Digital methods digitize the voice signal and use compression techniques to produce a very low-rate serial digital signal that can be modulated into a narrow band. Such digital modulation techniques are expected to meet the narrowbanding goal and provide some additional performance advantages.
New modulation techniques and protocols—including P25, TETRA, DMR, dPMR, and NXDN—have been developed to meet this need. All of these new methods must meet the requirements of the FCC’s Part 90 regulations and/or the regulations of the European Telecommunications Standards Institute (ETSI) standards such as TS-102 490 and TS-102-658 for LMR.
The most popular digital LMR technology, P25, is already in wide use in the U.S. with 12.5-kHz channels. Its frequency division multiple access (FDMA) method divides the assigned spectrum into 6.25-kHz or 12.5-kHz channels.
Phase I of the P25 project uses a four-symbol FSK (4FSK) modulation. Standard FSK, covered earlier, uses two frequencies or “tones” to achieve 1-bit/Hz. However, 4FSK is a variant that uses four frequencies to provide 2-bits/Hz efficiency. With this scheme the standard achieves a 9600-bit/s data rate in a 12.5-KHz channel. With 4FSK, the carrier frequency is shifted by ±1.8 kHz or ±600 Hz to achieve the four symbols.
In Phase 2, a compatible QPSK modulation scheme is used to achieve a similar data rate in a 6.25-kHz channel. The phase is shifted either ±45° or ±135° to get the four symbols. A unique demodulator has been developed to detect either the 4FSK or QPSK signal to recover the digital voice. Only different modulators on the transmit end are needed to make the transition from Phase 1 to Phase 2.
The most widespread digital LMR technology outside of the U.S. is TETRA, or Terrestrial Trunked Radio. This ETSI standard is universally used in Europe as well as in Africa, Asia, and Latin America. Its time division multiple access (TDMA) approach multiplexes four digital voice or data signals into a 25-kHz channel.
A single channel is used to support a digital stream of four time slots for the digital data for each subscriber. This is equivalent to four independent signals in adjacent 6.25-kHz channels. The modulation is π/4-DQPSK, and the data rate is 7.2 kbits/s per time slot.
Another ETSI standard, digital mobile radio (DMR), uses a 4FSK modulation scheme in a 12.5-kHz channel. It can achieve a 6.25-kHz channel equivalent in a 12.5-kHz channel by using two-slot TDMA. The voice is digitally coded with error correction, and the basic rate is 3.6 kbits/s. The data rate in the 12.5-kHz band is 9600 kbits/s.
A similar technology is dPMR, or digital private mobile radio standard. This ETSI standard also uses a 4FSK modulation scheme, but the access is FDMA in 6.25-kHz channels. The voice coding rate is also 3.6 kbits/s with error correction.
LMR manufacturers Icom and Kenwood have developed NXDN, another standard for LMR. It is designed to operate in either 12.5- or 6.25-kHz channels using digital voice compression and a four-symbol FSK system. A channel may be selected to carry voice or data.
The basic data rate is 4800 bits/s. The access method is FDMA. NXDN and dPMR are similar, as they both use 4FSK and FDMA in 6.25-kHz channels. The two methods are not compatible, though, as the data protocols and other features are not the same.
Because all of these digital techniques are similar and operate in standard frequency ranges, Freescale Semiconductor was able to make a single-chip digital radio that includes the RF transceiver plus an ARM9 processor that can be programmed to handle any of the digital standards. The MC13260 system-on-a-chip (SoC) can form the basis of a handset radio for any one if not multiple protocols. For more, see “Chip Makes Two-Way Radio Easy.”
Another example of modulation techniques improving spectral efficiency and increasing data throughput in a given channel is a new technique from NovelSat called NS3 modulation. Satellites are positioned in an orbit around the equator about 22,300 miles from earth. This is called the geostationary orbit, and satellites in it rotate in synchronization with the earth so they appear fixed in place, making them a good signal relay platform from one place to another on earth.
Satellites carry several transponders that pick up the weak uplink signal from earth and retransmit it on a different frequency. These transponders are linear and have a fixed bandwidth, typically 36 MHz. Some of the newer satellites have 72-MHz channel transponders. With a fixed bandwidth, the data rate is somewhat fixed as determined by the modulation scheme and access methods.
The question is how one deals with the need to increase the data rate in a remote satellite as required by the ever increasing demand for more traffic capacity. The answer lies in simply creating and implementing a more spectrally efficient modulation method. That’s what NovelSat did. Its NS3 modulation method increases bandwidth capacity up to 78%.
That level of improvement comes from a revised version of APSK modulation covered earlier. One commonly used satellite transmission standard, DVB-S2, is a single carrier (typically L-band, 950 to 1750 MHz) that can use QPSK, 8PSK, 16APSK, and 32APSK modulation with different forward error correction (FEC) schemes. The most common application is video transmission.
NS3 improves on DVB-S2 by offering 64APSK with multiple amplitude and phase symbols to improve efficiency. Also included is low density parity check (LDPC) coding. This combination provides a maximum data rate of 358 Mbits/s in a 72-MHz transponder. Because the modulation is APSK, the TWT PAs don’t have to be backed off to preserve perfect linearity. As a result, they can operate at a higher power level and achieve the higher data rate with a lower CNR than DVB-S2. NovelSat offers its NS1000 modulator and NS2000 demodulator units to upgrade satellite systems to NS3. In most applications, NS3 provides a data rate boost over DVB-S2 for a given CNR.
Established in 2000, the Soukacatv.com (DSW) main products are modulators both in digital and analog modulators, amplifier and combiner. We are the leading communication supplier in manufacturing the headend system in China. Our 16 in 1 and 24 in 1 now are the most popular products all over the world. For more, please access to https://www.soukacatv.com/.
Source: electronicdesign
2018年12月20日星期四
Digital Amplitude Modulation and Digital Frequency Modulation | Soukacatv.com
Though based on the same
concepts, digital modulation waveforms look quite different from their analog
counterparts.
Though far from extinct,
analog modulation is simply incompatible with a digital world. We no longer
focus our efforts on moving analog waveforms from one place to another. Rather,
we want to move data: wireless networking, digitized audio signals, sensor measurements,
and so forth. To transfer digital data, we use digital modulation.
We have to be careful,
though, with this terminology. “Analog” and “digital” in this context refer to
the type of information being transferred, not to the basic characteristics of
the actual transmitted waveforms. Both analog and digital modulation use
smoothly varying signals; the difference is that an analog-modulated signal is
demodulated into an analog baseband waveform, whereas a digitally modulated
signal consists of discrete modulation units, called symbols, that are interpreted
as digital data.
There are analog and digital versions of the
three modulation types. Let’s start with amplitude and frequency.
HDMI Encoder Modulator,16in1 Digital Headend, HD RF Modulator at SOUKA https://www.soukacatv.com/.
HDMI Encoder Modulator,16in1 Digital Headend, HD RF Modulator at SOUKA https://www.soukacatv.com/.
Digital Amplitude
Modulation
This type of modulation
is referred to as amplitude shift keying (ASK). The most basic case is “on-off
keying” (OOK), and it corresponds almost directly to the mathematical
relationship discussed in the page dedicated to analog amplitude modulation: If
we use a digital signal as the baseband waveform, multiplying the baseband and
the carrier results in a modulated waveform that is normal for logic high and
“off” for logic low. The logic-high amplitude corresponds to the modulation
index.
Time Domain
The following plot shows
OOK generated using a 10 MHz carrier and a 1 MHz digital clock signal. We’re
operating in the mathematical realm here, so the logic-high amplitude (and the
carrier amplitude) is simply dimensionless “1”; in a real circuit you might
have a 1 V carrier waveform and a 3.3 V logic signal.
You may have noticed one inconsistency
between this example and the mathematical relationship discussed in the
[[Amplitude Modulation]] page: we didn’t shift the baseband signal. If you’re
dealing with a typical DC-coupled digital waveform, no upward shifting is
necessary because the signal remains in the positive portion of the y-axis.
Frequency Domain
Here is the
corresponding spectrum:
Compare this to the spectrum for amplitude
modulation with a 1 MHz sine wave:
Most of the spectrum is the same—a spike at
the carrier frequency (fC)
and a spike at fC plus
the baseband frequency and fC minus
the baseband frequency. However, the ASK spectrum also has smaller spikes that
correspond to the 3rd and 5th harmonics: The fundamental frequency (fF) is 1 MHz, which means
that the 3rd harmonic (f3)
is 3 MHz and the 5th harmonic (f5)
is 5 MHz. So we have spikes at fC plus/minus
fF, f3, and f5. And actually, if you
were to expand the plot, you would see that the spikes continue according to
this pattern.
This makes perfect
sense. A Fourier transform of a square wave consists of a sine wave at the
fundamental frequency along with decreasing-amplitude sine waves at the odd
harmonics, and this harmonic content is what we see in the spectrum shown
above.
This discussion leads us
to an important practical point: abrupt transitions associated with digital
modulation schemes produce (undesirable) higher-frequency content. We have to
keep this in mind when we consider the actual bandwidth of the modulated signal
and the presence of frequencies that could interfere with other devices.
Digital Frequency Modulation
This type of modulation
is called frequency shift keying (FSK). For our purposes it is not necessary to
consider a mathematical expression of FSK; rather, we can simply specify that
we will have frequency f1 when
the baseband data is logic 0 and frequency f2 when the baseband data is logic 1.
Time Domain
One method of generating
the ready-for-transmission FSK waveform is to first create an analog baseband
signal that switches between f1 and
f2 according to
the digital data. Here is an example of an FSK baseband waveform with f1 = 1 kHz and f2 = 3 kHz. To ensure
that a symbol is the same duration for logic 0 and logic 1, we use one 1 kHz
cycle and three 3 kHz cycles.
The baseband waveform is then shifted
(using a mixer) up to the carrier frequency and transmitted. This approach is
particularly handy in software-defined-radio systems: the analog baseband
waveform is a low-frequency signal, and thus it can be generated mathematically
then introduced into the analog realm by a DAC. Using a DAC to create the
high-frequency transmitted signal would be much more difficult.
A more conceptually
straightforward way to implement FSK is to simply have two carrier signals with
different frequencies (f1 and
f2); one or the other
is routed to the output depending on the logic level of the binary data. This
results in a final transmitted waveform that switches abruptly between two
frequencies, much like the baseband FSK waveform above except that the
difference between the two frequencies is much smaller in relation to the
average frequency. In other words, if you were looking at a time-domain plot,
it would be difficult to visually differentiate the f1 sections from the f2 sections because the difference between f1 and f2 is only a tiny
fraction of f1 (or
f2).
Frequency Domain
Let’s look at the
effects of FSK in the frequency domain. We’ll use our same 10 MHz carrier
frequency (or average carrier frequency in this case), and we’ll use ±1 MHz as
the deviation. (This is unrealistic, but convenient for our current purposes.)
So the transmitted signal will be 9 MHz for logic 0 and 11 MHz for logic 1.
Here is the spectrum:
Note that there is no energy at the
“carrier frequency.” This is not surprising, considering that the modulated
signal is never at 10 MHz. It is always at either 10 MHz minus 1 MHz or 10 MHz
plus 1 MHz, and this is precisely where we see the two dominant spikes: 9 MHz
and 11 MHz.
But what about the other
frequencies present in this spectrum? Well, FSK spectral analysis is not
particularly straightforward. We know that there will be additional Fourier
energy associated with the abrupt transitions between frequencies. It turns out
that FSK results in a sinc-function type of spectrum for each frequency, i.e.,
one is centered on f1 and
the other is centered on f2.
These account for the additional frequency spikes seen on either side of the
two dominant spikes.
Summary
- Digital
amplitude modulation involves varying the amplitude of a carrier wave in
discrete sections according to binary data.
- The
most straightforward approach to digital amplitude modulation is on-off
keying.
- With
digital frequency modulation, the frequency of a carrier or a baseband
signal is varied in discrete sections according to binary data.
- If we
compare digital modulation to analog modulation, we see that the abrupt
transitions created by digital modulation result in additional energy at
frequencies farther from the carrier.
Established in 2000, the Soukacatv.com (DSW)
main products are modulators both in digital and analog modulators, amplifier
and combiner. We are the leading communication supplier in manufacturing the
headend system in China. Our 16 in 1 and 24 in 1 now are the most popular
products all over the world. For more, please access to https://www.soukacatv.com/.
Source: allaboutcircuits
2018年12月18日星期二
Brief Introduction about the Definition and Characteristics of Digital Signal | Soukacatv.com
A digital signal refers to an electrical signal that is converted into a pattern of bits. Unlike an analog signal, which is a continuous signal that contains time-varying quantities, a digital signal has a discrete value at each sampling point. The precision of the signal is determined by how many samples are recorded per unit of time. For example, the illustration below shows an analog pattern (represented as the curve) alongside a digital pattern (represented as the discrete lines).
A digital signal is easily represented by a computer because each sample can be defined with a series of bits that are either in the state 1 (on) or 0 (off). Digital signals can be compressed and can include additional information for error correction.
HDMI Encoder Modulator,16in1 Digital Headend,HD RF Modulator at SOUKA https://www.soukacatv.com/.
Characteristics of Digital Signals
1. Bit interval
It is the time required to send one single bit
2. Bit rate
(i) It refers to the number of bit intervals in one second.
(ii) Therefore bit rate is the number of bits sent in one second as shown in fig.
(iii)Bit rate is expressed in bits per second (bps).
(iv)Other units used to express bit rate are Kbps, Mbps and Gbps.
1 kilobit per second (Kbps) = 1,000 bits per second
1 Megabit per second (Mbps) = 1,000,000 bits per second
1 Gigabit per second (Gbps) = 1,000,000,000 bits per second
Advantages of Digital Signals
Digital Data - Digital transmission certainly has the advantage where binary computer data is being transmitted. The equipment required to convert digital data to analog format and transmitting the digital bit streams over an analog network can be expensive, susceptible to failure, and can create errors in the information.
Compression - Digital data can be compressed relatively easily, thereby increasing the efficiency of transmission. As a result, substantial volumes of voice, data, video and image information can be transmitted using relatively little raw bandwidth.
Security - Digital systems offer better security. While analog systems offer some measure of security through the scrambling of several frequencies. Scrambling is fairly simple to defeat. Digital information, on the other hand, can be encrypted to create the appearance of a single, pseudorandom bit stream. Thereby, the true meaning of individual bits, sets of bits, or the total bit stream cannot be determined without having the key to unlock the encryption algorithm employed.
Quality - Digital transmission offers improved error performance (quality) as compared to analog. This is due to the devices that boost the signal at periodic intervals in the transmission system in order to overcome the effects of attenuation. Additionally, digital networks deal more effectively with noise, which always is present in transmission networks.
Cost - The cost of the computer components required in digital conversion and transmission has dropped considerably, while the ruggedness and reliability of those components has increased over the years.
Upgradeability - Since digital networks are comprised of computer (digital) components, they are relatively easy to upgrade. Such upgrading can increase bandwidth, reduces the incidence of error and enhance functional value. Some upgrading can be effected remotely over a network, eliminating the need to dispatch expensive technicians for that purpose.
Management - Generally speaking, digital networks can be managed much more easily and effectively due to the fact that such networks consist of computerized components. Such components can sense their own level of performance, isolate and diagnose failures, initiate alarms, respond to queries, and respond to commands to correct any failure. Further, the cost of these components continues to drop.
Established in 2000, the Soukacatv.com (DSW) main products are modulators both in digital and analog modulators, amplifier and combiner. We are the leading communication supplier in manufacturing the headend system in China. Our 16 in 1 and 24 in 1 now are the most popular products all over the world. For more, please access to https://www.soukacatv.com/.
Source: ecomputernotes
A digital signal is easily represented by a computer because each sample can be defined with a series of bits that are either in the state 1 (on) or 0 (off). Digital signals can be compressed and can include additional information for error correction.
HDMI Encoder Modulator,16in1 Digital Headend,HD RF Modulator at SOUKA https://www.soukacatv.com/.
Characteristics of Digital Signals
1. Bit interval
It is the time required to send one single bit
2. Bit rate
(i) It refers to the number of bit intervals in one second.
(ii) Therefore bit rate is the number of bits sent in one second as shown in fig.
(iii)Bit rate is expressed in bits per second (bps).
(iv)Other units used to express bit rate are Kbps, Mbps and Gbps.
1 kilobit per second (Kbps) = 1,000 bits per second
1 Megabit per second (Mbps) = 1,000,000 bits per second
1 Gigabit per second (Gbps) = 1,000,000,000 bits per second
Advantages of Digital Signals
Digital Data - Digital transmission certainly has the advantage where binary computer data is being transmitted. The equipment required to convert digital data to analog format and transmitting the digital bit streams over an analog network can be expensive, susceptible to failure, and can create errors in the information.
Compression - Digital data can be compressed relatively easily, thereby increasing the efficiency of transmission. As a result, substantial volumes of voice, data, video and image information can be transmitted using relatively little raw bandwidth.
Security - Digital systems offer better security. While analog systems offer some measure of security through the scrambling of several frequencies. Scrambling is fairly simple to defeat. Digital information, on the other hand, can be encrypted to create the appearance of a single, pseudorandom bit stream. Thereby, the true meaning of individual bits, sets of bits, or the total bit stream cannot be determined without having the key to unlock the encryption algorithm employed.
Quality - Digital transmission offers improved error performance (quality) as compared to analog. This is due to the devices that boost the signal at periodic intervals in the transmission system in order to overcome the effects of attenuation. Additionally, digital networks deal more effectively with noise, which always is present in transmission networks.
Cost - The cost of the computer components required in digital conversion and transmission has dropped considerably, while the ruggedness and reliability of those components has increased over the years.
Upgradeability - Since digital networks are comprised of computer (digital) components, they are relatively easy to upgrade. Such upgrading can increase bandwidth, reduces the incidence of error and enhance functional value. Some upgrading can be effected remotely over a network, eliminating the need to dispatch expensive technicians for that purpose.
Management - Generally speaking, digital networks can be managed much more easily and effectively due to the fact that such networks consist of computerized components. Such components can sense their own level of performance, isolate and diagnose failures, initiate alarms, respond to queries, and respond to commands to correct any failure. Further, the cost of these components continues to drop.
Established in 2000, the Soukacatv.com (DSW) main products are modulators both in digital and analog modulators, amplifier and combiner. We are the leading communication supplier in manufacturing the headend system in China. Our 16 in 1 and 24 in 1 now are the most popular products all over the world. For more, please access to https://www.soukacatv.com/.
Source: ecomputernotes
2018年12月17日星期一
Reading About the Analog vs. Digital Signals | Soukacatv.com
Overview
We live in an analog world. There are an infinite amount of colors to paint an object (even if the difference is indiscernible to our eye), there are an infinite number of tones we can hear, and there are an infinite number of smells we can smell. The common theme among all of these analog signals is their infinite possibilities.
Digital signals and objects deal in the realm of the discrete or finite, meaning there is a limited set of values they can be. That could mean just two total possible values, 255, 4,294,967,296, or anything as long as it’s not ∞ (infinity).
Working with electronics means dealing with both analog and digital signals, inputs and outputs. Our electronics projects have to interact with the real, analog world in some way, but most of our microprocessors, computers, and logic units are purely digital components. These two types of signals are like different electronic languages; some electronics components are bi-lingual, others can only understand and speak one of the two.
In this tutorial, we’ll cover the basics of both digital and analog signals, including examples of each. We’ll also talk about analog and digital circuits, and components.
HDMI Encoder Modulator,16in1 Digital Headend, HD RF Modulator at SOUKA https://www.soukacatv.com/.
Suggested Reading
The concepts of analog and digital stand on their own, and don’t require a lot of previous electronics knowledge. That said, if you haven’t already, you should peek through some of these tutorials:
• Voltage, Current, Resistance and Ohm’s Law
• What is a Circuit
• And some mathematics concepts: reading graphs, and understanding the difference between finite and infinite sets.
Analog Signals
Define: Signals
Before going too much further, we should talk a bit about what a signal actually is, electronic signals specifically (as opposed to traffic signals, albums by the ultimate power-trio, or a general means for communication). The signals we’re talking about are time-varying “quantities” which convey some sort of information. In electrical engineering the quantity that’s time-varying is usually voltage (if not that, then usually current). So when we talk about signals, just think of them as a voltage that’s changing over time.
Signals are passed between devices in order to send and receive information, which might be video, audio, or some sort of encoded data. Usually the signals are transmitted through wires, but they could also pass through the air via radio frequency (RF) waves. Audio signals, for example might be transferred between your computer’s audio card and speakers, while data signals might be passed through the air between a tablet and a WiFi router.
Analog Signal Graphs
Because a signal varies over time, it’s helpful to plot it on a graph where time is plotted on the horizontal, x-axis, and voltage on the vertical, y-axis. Looking at a graph of a signal is usually the easiest way to identify if it’s analog or digital; a time-versus-voltage graph of an analog signal should be smooth and continuous.
While these signals may be limited to a range of maximum and minimum values, there are still an infinite number of possible values within that range. For example, the analog voltage coming out of your wall socket might be clamped between -120V and +120V, but, as you increase the resolution more and more, you discover an infinite number of values that the signal can actually be (like 64.4V, 64.42V, 64.424V, and infinite, increasingly precise values).
Example Analog Signals
Video and audio transmissions are often transferred or recorded using analog signals. The composite video coming out of an old RCA jack, for example, is a coded analog signal usually ranging between 0 and 1.073V. Tiny changes in the signal have a huge effect on the color or location of the video.
An analog signal representing one line of composite video data.
Pure audio signals are also analog. The signal that comes out of a microphone is full of analog frequencies and harmonics, which combine to make beautiful music.
Digital Signals
Digital signals must have a finite set of possible values. The number of values in the set can be anywhere between two and a-very-large-number-that’s-not-infinity. Most commonly digital signals will be one of two values – like either 0V or 5V. Timing graphs of these signals look like square waves.
Or a digital signal might be a discrete representation of an analog waveform. Viewed from afar, the wave function below may seem smooth and analog, but when you look closely there are tiny discrete steps as the signal tries to approximate values:
That’s the big difference between analog and digital waves. Analog waves are smooth and continuous, digital waves are stepping, square, and discrete.
Example Digital Signals
Not all audio and video signals are analog. Standardized signals like HDMI for video (and audio) and MIDI, I2S, or AC'97for audio are all digitally transmitted.
Most communication between integrated circuits is digital. Interfaces like serial, I2C, and SPI all transmit data via a coded sequence of square waves.
Serial peripheral interface (SPI) uses many digital signals to transmit data between devices.
Analog and Digital Circuits
Analog Electronics
Most of the fundamental electronic components – resistors, capacitors, inductors, diodes, transistors, and operational amplifiers – are all inherently analog. Circuits built with a combination of solely these components are usually analog.
Analog circuits are usually complex combinations of op amps, resistors, caps, and other foundational electronic components. This is an example of a class B analog audio amplifier.
Analog circuits can be very elegant designs with many components, or they can be very simple, like two resistors combining to make a voltage divider. In general, though, analog circuits are much more difficult to design than those which accomplish the same task digitally. It takes a special kind of analog circuit wizard to design an analog radio receiver, or an analog battery charger; digital components exist to make those designs much simpler.
Analog circuits are usually much more susceptible to noise (small, undesired variations in voltage). Small changes in the voltage level of an analog signal may produce significant errors when being processed.
Digital Electronics
Digital circuits operate using digital, discrete signals. These circuits are usually made of a combination of transistors and logic gates and, at higher levels, microcontrollers or other computing chips. Most processors, whether they’re big beefy processors in your computer, or tiny little microcontrollers, operate in the digital realm.
Digital circuits make use of components like logic gates, or more complicated digital ICs (usually represented by rectangles with labeled pins extending from them).
Digital circuits usually use a binary scheme for digital signaling. These systems assign two different voltages as two different logic levels – a high voltage (usually 5V, 3.3V, or 1.8V) represents one value and a low voltage (usually 0V) represents the other.
Although digital circuits are generally easier to design, they do tend to be a bit more expensive than an equally tasked analog circuit.
Analog and Digital Combined
It’s not rare to see a mixture of analog and digital components in a circuit. Although microcontrollers are usually digital beasts, they often have internal circuitry which enables them to interface with analog circuitry (analog-to-digital converters, pulse-width modulation, and digital-to-analog converters. An analog-to-digital converter (ADC) allows a microcontroller to connect to an analog sensor (like photocells or temperature sensors), to read in an analog voltage. The less common digital-to-analog converter allows a microcontroller to produce analog voltages, which is handy when it needs to make sound.
Resources and Going Further
Now that you know the difference between analog and digital signals, we’d suggest checking out the Analog to Digital Conversion tutorial. Working with microcontrollers, or really any logic-based electronics, means working in the digital realm most of the time. If you want to sense light, temperature, or interface a microcontroller with a variety of other analog sensors, you’ll need to know how to convert the analog voltage they produce into a digital value.
Also, consider reading our Pulse Width Modulation (PWM) tutorial. PWM is a trick microcontrollers can use to make a digital signal appear to be analog.
Here are some other subjects which deal heavily with digital interfaces:
• Binary
• Logic Levels
• Serial Communication
• SPI Communication
• I2C Communication
• IR Communication
Or, if you’d like to delve further into the analog realm, consider checking out these tutorials:
• Voltage Dividers
• Resistors
• Diodes
• Capacitors
• Transistors
Established in 2000, the Soukacatv.com (DSW) main products are modulators both in digital and analog modulators, amplifier and combiner. We are the leading communication supplier in manufacturing the headend system in China. Our 16 in 1 and 24 in 1 now are the most popular products all over the world. For more, please access to https://www.soukacatv.com/.
Source: learn.sparkfun
订阅:
博文 (Atom)